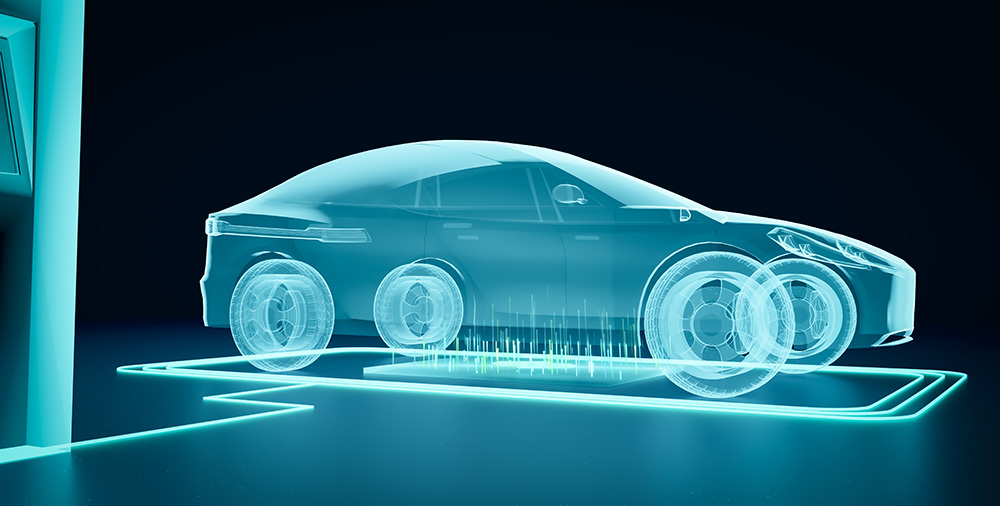
Ditch the plug! Make wireless EV charging a reality now!
Unique components ensure safety, efficiency and reliability.
Imagine an EV charging experience that’s as seamless and intuitive as parking your car—just “park, charge, and go.” For the end-user, the appeal is clear:
- No more heavy cables
- No fumbling with connectors
- No exposure to potentially dirty or damaged charging equipment
EV owners envision pulling into their garage, a designated parking spot, or a public charging station and effortlessly charging their vehicle without ever leaving the driver’s seat. This convenience makes daily charging more user-friendly and enhances safety by removing physical connectors altogether.
Understanding this user-centric vision is crucial for wireless EV charging system designers. By delivering efficient, reliable, and fast wireless charging, designers can unlock a game-changing benefit that combines ease, comfort, and peace of mind for EV drivers.
While current wireless EV chargers can supply up to 20 kW to charge batteries in four to six hours, future wireless chargers will deliver 100 kW and be able to increase battery charge state by 50 percent in under 20 minutes.1
Wireless charging stations must be fast, safe, efficient, and reliable to accelerate adoption.
This article explores the technical considerations and innovative approaches required to achieve this experience, ensuring wireless charging solutions meet performance and user expectations in the ever-evolving EV landscape. It presents four components that address the essential needs to create designs that ensure charger circuit protection, safety monitoring, and fast, efficient power delivery.
Wireless charger description
A wireless charger is an AC-AC converter that converts 50/60 Hz power to power in the frequency range of 130 kHz. Resonant frequency depends on topologies and Power semi conductor technology (Si/SiC/GaN). Power delivery can be up to 20 kW. Figure 1 illustrates a wireless charger and its load, the EV. The major power and control circuit blocks in the charger and the vehicle are also defined.
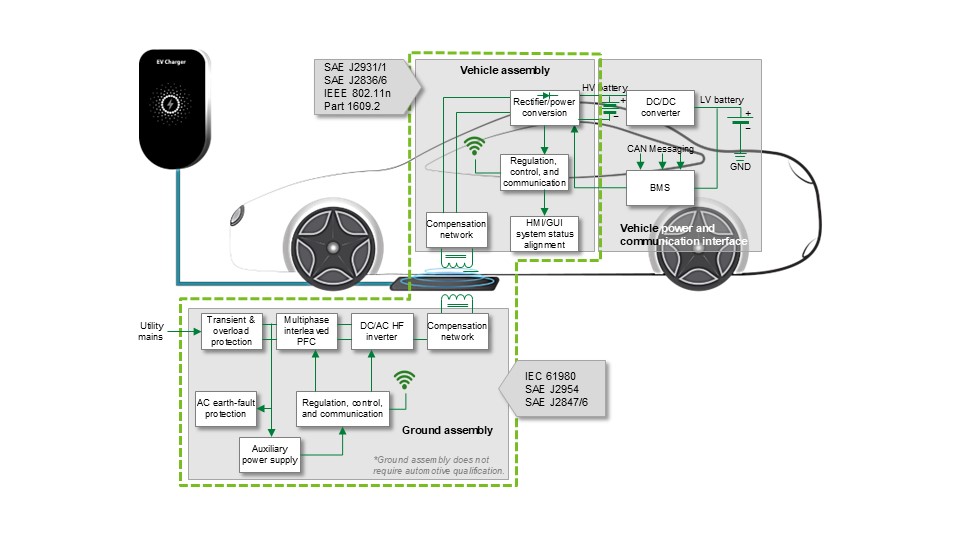
Safety and reliability considerations include overcurrent protection, overvoltage protection, overtemperature, and ground current monitoring. Optimizing efficiency requires designing with low power loss components. Figure 2 illustrates components that provide circuit protection and high efficiency for the circuits of a typical wireless charger design. The sensors supply temperature monitoring and enclosure access protection.
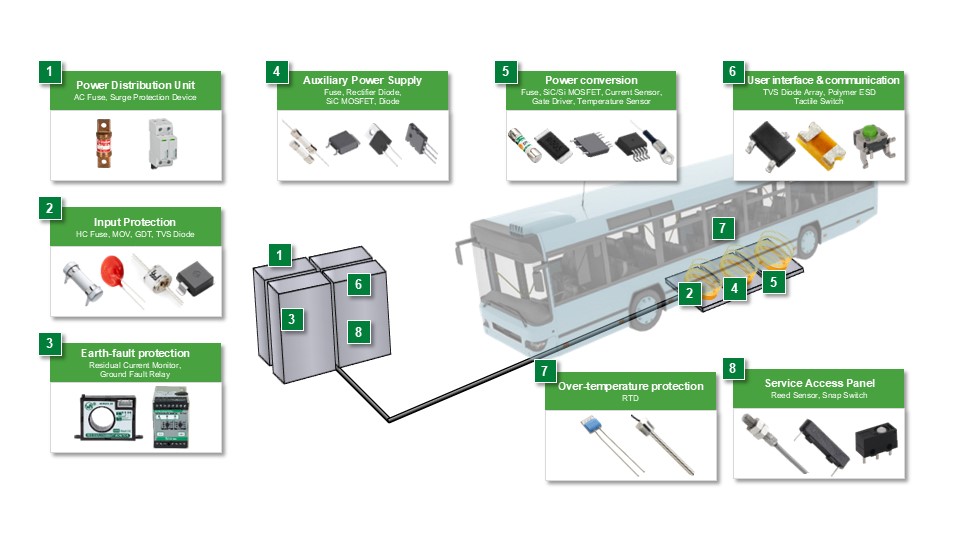
Figures 3 and 4 show an example wireless charger in a more detailed block diagram. The adjacent table in Figure 3 lists the components that equip the charger with protection from electrical hazards. Figure 4 primarily shows the components that produce efficiency and critical sensing.
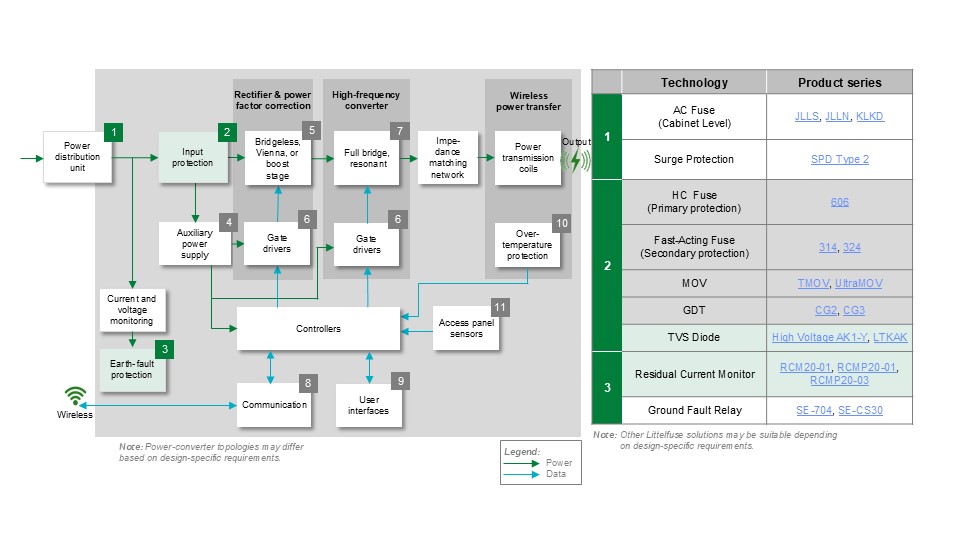
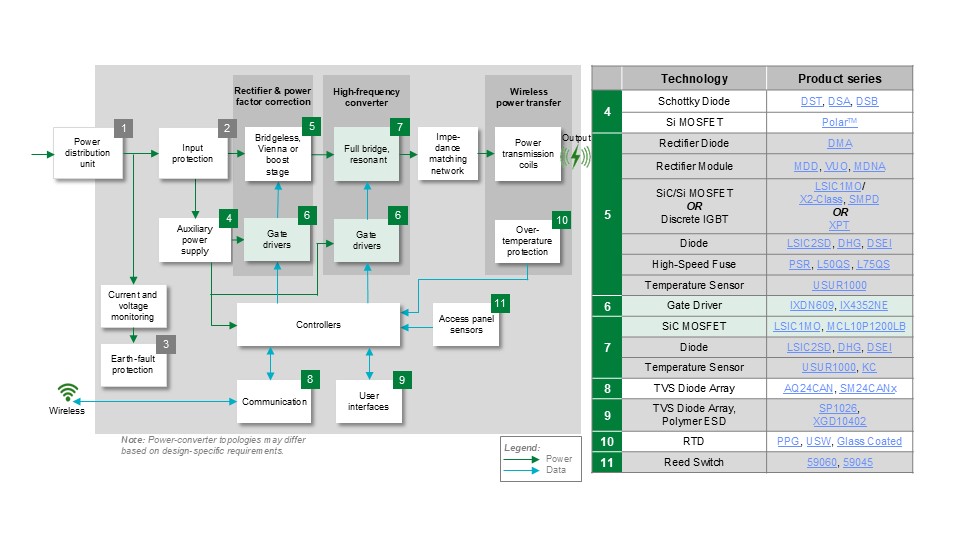
Circuit protection and safety components
The Input Protection circuit houses the main overcurrent and overtemperature protection components. Recommended components include a high current fuse for the power delivery circuitry and a fast-acting fuse to protect the low power Auxiliary Power Supply and the control circuitry. A metal oxide varistor (MOV) in series with a gas discharge tube absorbs overvoltage transients. Overvoltage transients result from lightning which can induce a voltage surge on the AC input lines. In addition, electric loads switching on and off can induce AC line voltage surges.
A special component that can capture portions of a voltage transient that has passed through the MOV and gas discharge tube is a transient voltage suppressor (TVS) diode. TVS diodes have lower clamping voltages, and they operate much faster than MOV devices. The special diodes can ensure protection of downstream circuitry. They can absorb a one kA pulse and respond to a transient in under one nanosecond. TVS diodes can provide protection from electrostatic discharge (ESD) through-the-air strikes of up to 15 kV and from direct contact discharges up to 8 kV. Bi-directional models and models that are less than one tenth the size of traditional discrete solutions are available. TVS diodes can have axial lead or surface mount form factors. Figure 5 shows a TVS diode and its functional diagram, using the AK1-Y Series TVS Diode from Littelfuse as an example. This component will dispense the necessary protection from both ESD and other transients to avoid damage to semiconductor circuitry in the wireless charger.
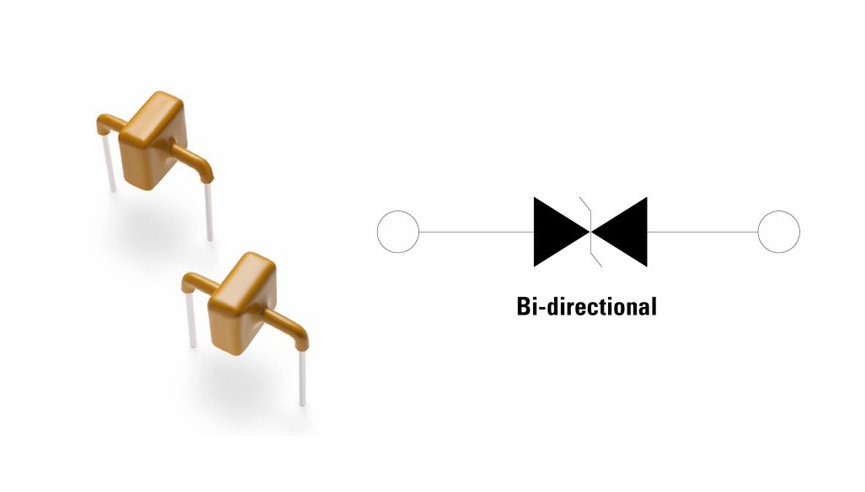
With systems such as wireless EV chargers, monitoring ground currents is essential for the protection of personnel. The Earth-Fault Protection circuit performs the ground current monitoring function. Littelfuse offers new residual current monitors for this circuit that detect both AC and DC ground fault currents. The new series, the RCMP20 Residual Current Monitor Series for Mode 2 and Mode 3 wireless charging stations, offers the largest current transformer aperture to support higher AC charging currents. The residual current monitors have sensitive, typical trip thresholds of 4.5 mA DC and 22 mA AC. Furthermore, the monitors utilize integrated conductors with higher cross-sectional areas to provide better thermal management and reduce the rise in the printed circuit board (PCB) temperature. The result is a more compact and reliable design that does not compromise performance. In addition, the monitors have high immunity to electromagnetic interference (EMI), which improves charger circuit reliability and minimizes false circuit trips. The monitors can be mounted either horizontally or vertically to allow designers flexibility to optimize space utilization. Figure 6 displays the models in the Residual Current Monitor series. (View the video.)
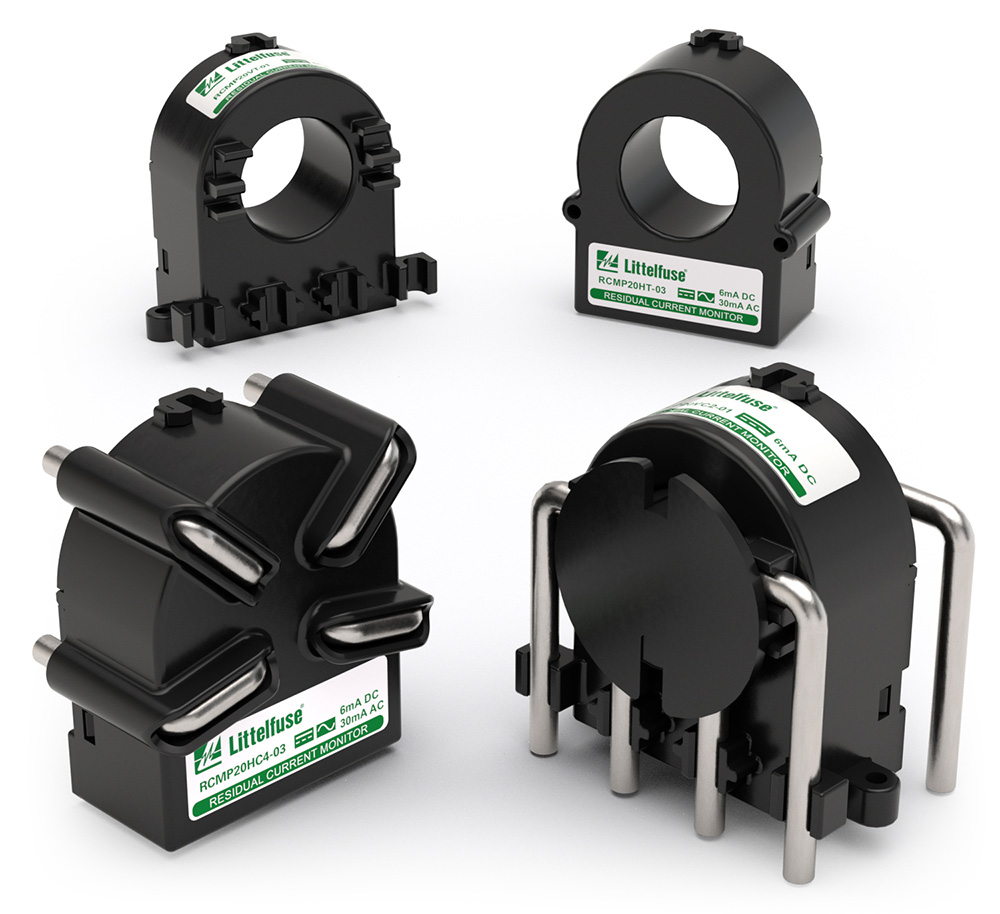
Components for maximizing efficiency and reliability
Systems, such as wireless charging systems, consume a substantial amount of power. Optimizing a design for efficiency reduces power consumption and utility costs and reduces heat buildup. Reduced generated heat lowers the internal temperature rise in the system and enhances system reliability. The use of two components in the power delivery circuitry can contribute to higher efficiency and greater reliability. The two components are gate drivers and SiC MOSFETs.
Gate drivers control the Power SiC MOSFETs and the IGBTs in the Bridgeless, Vienna, or Boost Rectifier and the Full Bridge, Resonant High-Frequency Converter circuits. The drivers have separate 9 A source and sink outputs, which enable programmable turn-on and turn-off timing while minimizing switching losses. An internal negative charge regulator provides a selectable negative gate drive bias for improved dV/dt immunity and faster turn-off. The gate drivers minimize switching times with turn-on and turn-off propagation delay times of typically 70 and 65 nanoseconds. The typical value for rise time and fall time outputs is ten nanoseconds.
To ensure robust operation, the gate drivers have desaturation detection circuitry which senses a SiC MOSFET overcurrent condition and initiates a soft turn off. This circuit prevents a potentially damaging dV/dt event. Additional protection features include UVLO detection and thermal shutdown. Figure 7 illustrates theLittelfuse IX4352NE SiC MOSFET and IGBT Driver IC, a high-speed gate driver with features that provide reliable control of a SiC MOSFET.
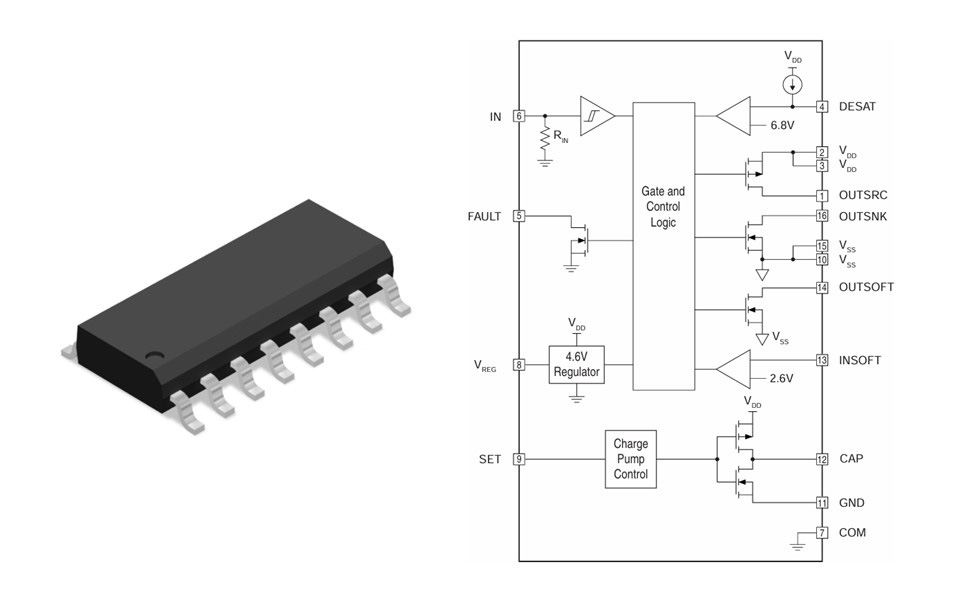
High-power SiC MOSFETs drive the power transmission coils. Half-bridge packages have a Drain-Source voltage of 1200 V and a drain current of up to 19.5 A. Along with delivering high power, the MOSFETs minimize on-state power consumption with a typical RDS(ON) of a low 160 mΩ. SiC MOSFETs have low switching power losses due to a typical low gate charge, short turn-on, and turn-off delay times, and current rise and fall times.
A DCB-based isolated package improves thermal resistance and power handling capability. An advanced topside cooled package simplifies thermal management. The Littelfuse half-bridge SiC MOSFET MCL10P1200LB Series, shown in Figure 8, yields high efficiency with advanced packaging to reduce component count and to optimize for high reliability.
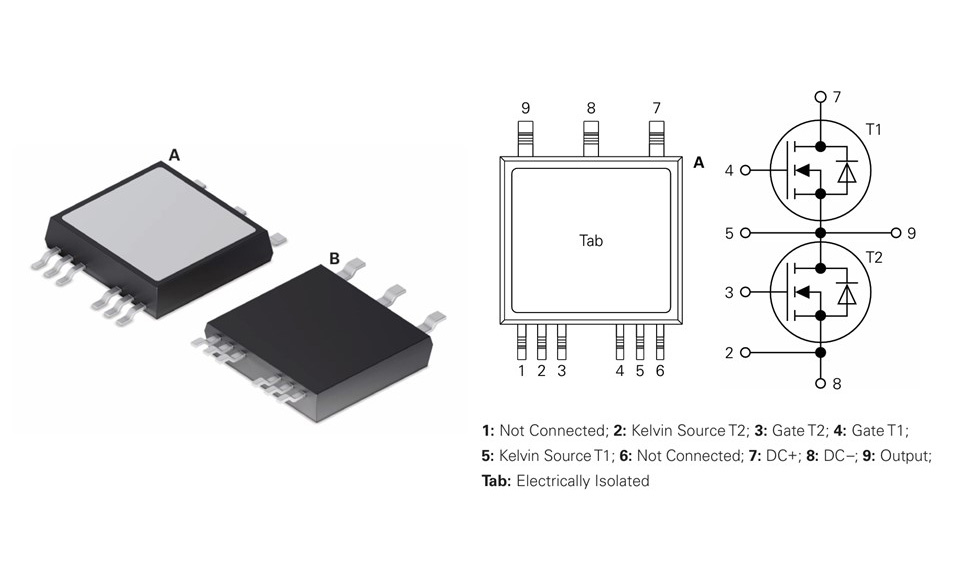
Collaborate with experts for a reliable wireless charging solution
Protection against electrical hazards such as overcurrent, overvoltage, ESD, and overtemperature is critical for ensuring reliable operation. The four recommended components described in the preceding paragraphs enable designers to develop robust, safe, and reliable wireless EV charging stations.
To develop a robust and efficient product, designers should consider utilizing the component manufacturers’ application engineers to save design time and compliance costs. The application engineers can help with the following:
- Selection of cost-effective protection, sensing, and high-efficiency components
- Knowledge of the applicable safety standards
- Littelfuse can perform pre-compliance testing to avoid compliance test failures and save on project delays and added costs for multiple compliance test cycles.
Collaborating with the component manufacturer’s application engineers and using the recommended components will help to produce robust, reliable, and efficient wireless EV charging solutions.
To learn more about circuit protection, sensing, and power management solutions for wireless EV charging design, download the guide, Supercharged Solutions for EV Charging Stations, courtesy of Littelfuse, Inc.
Contact Littelfuse for more information on making your wireless charging system design safe, efficient and reliable.
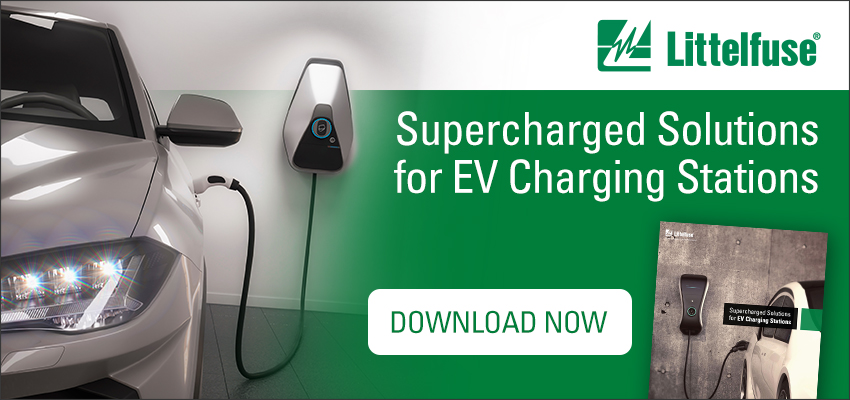
References:
1L. Blain. World’s fastest wireless EV charger unlocks 100 kW parking spots. New Atlas. March 18, 2024.